
J.
Physiol.
(1971),
216,
pp.
181-200
181
With
4
text-figurew
Printed
in
Great
Britain
THE
HEAT
OF
SHORTENING
DURING
THE
PLATEAU
OF
TETANIC
CONTRACTION
AND
AT
THE
END
OF
RELAXATION
BY
X.
AUBERT
AND
J.
LEBACQ*
From
the
Laboratoire
de
Physiologie
G04nerale,
Universitet
Catholique
de
Louvain,
Belgium
(Received
30
December
1970)
SUMMARY
1.
The
net
thermal
effect
of
shortening
on
the
heat
production
of
the
sartorius
muscle
of
Rana
temporaria
has
been
studied
at
00
C
in
tetanic
contractions
of
either
1-2
or
2-0
see
analysed
up
to
the
end
of
relaxation.
2.
Three
types
of
contractions
have
been
compared:
isometric
at
the
standard
length
(IO);
isometric
at
a
shorter
length
(lo
minus
4-27
mm);
and
rapid
shortening
at
constant
velocity
between
those
two
lengths
during
the
plateau
of
tetanic
activity,
the
work
being
collected
by
an
ergometer
of
the
Levin-Wyman
type.
After
the
end
of
the
shortening,
stimulation
was
continued
long
enough
to
allow
a
complete
recovery
of
tension
before
relaxation
started.
3.
In
those
conditions,
shortening
heat
appears
clearly
by
comparison
with
the
isometric
contractions,
and
most
of
its
effect
persists
up
to
the
end
of
relaxation.
However,
a
reduction
of
the
net
thermal
effect,
of
the
order
of
15-25
%,
is
observed
during
relaxation.
4.
The
net
supplement
of
heat
is
the
same
whether
the
shortening
occurs
in
the
beginning
(0.4
see)
or
towards
the
end
(1-2
see)
of
the
con-
traction
period;
the
reduction
of
the
effect
during
relaxation
is
more
important
in
the
pelvic
part
than
in
the
tibial
part
of
the
muscle.
5.
This
small
reduction
of
the
thermal
effect
of
the
shortening
is
fully
accounted
for
by
the
fact
that
the
tension
redeveloped
after
the
end
of
the
movement
remains
always
below
the
tension
developed
in
a
tetanus
at
the
same
final
length,
but
isometric
from
the
start.
6.
As
the
greater
part
of
the
net
thermal
effect
of
the
shortening
persists
at
the
end
of
relaxation,
shortening
heat
cannot
be
part
of
a
cyclic
process
reversed
during
relaxation;
it
must
derive
from
some
chemical
source
of
energy.
*
Aspirant
du
F.N.R.S.
(Belgium).

X.
A
UBERT
AND
J.
LEBACQ
INTRODUCTION
According
to
Fenn
(1923),
when
a
muscle
shortens
during
contraction
it
liberates
energy
in
excess
of
isometric
heat,
mainly
in
the
form
of
mechanical
work
if
a
load
is
moved,
but
partly
also
as
a
supplement
of
heat
production.
Hill
(1938,
1949)
showed
that
this
supplement
measured
during
contraction
was
proportional
to
the
change
of
length
and
called
it
'heat
of
shortening';
later
he
found
that
it
was
also
a
function
of
the
dis-
placed
load
(Hill,
1964a).
Biochemical
studies
made
under
various
experimental
conditions
detected
chemical
reactions
yielding
enough
energy
to
account
for
the
work
and
for
a
part
at
least
of
the
heat
of
activation
and
of
maintenance
of
tension,
but
they
systematically
failed-
to
account
for
the
heat
of
shortening
(Mommaerts,
Seraydarian
&
Marechal,
1962;
Cain,
Infante
&
Davies,
1962;
Carlson,
Hardy
&
Wilkie,
1963;
Mar6chal,
1964;
Davies,
Kushmerick
&
Larson,
1967;
Kushmerick,
Larson
&
Davies,
1969).
In
the
past,
shortening
heat
has
usually
been
measured
during
the
course
of
the
contraction,
the
relaxation
phase
being
excluded,
whereas
most
biochemical
analyses
followed
a
whole
series
of
contraction-relaxa-
tion
cycles.
The
simplest
explanation
for
the
absence
of
a
chemical
equi-
valent
of
shortening
heat
has
been
suggested
by
Carlson
et
al.
(1963).
Measuring
total
heat
and
chemical
transformation
in
the
same
muscle
after
a
series
of
isotonic
twitches,
they
did
not
find
in
those
conditions
any
net
thermal
effect
of
the
shortening.
According
to
these
authors,
the
process
responsible
for
the
heat
of
shortening
could
be
reversed
during
relaxation,
so
that
the
net
effect
of
the
movement
would
be
nil,
except
for
the
per-
formance
of
work.
They
concluded,
'Hill
originally
described
shortening
heat
for
a
tetanic
contraction.
It
is
doubtful
whether
in
this
case
either,
shorten-
ing
heat
contributes
to
the
net
energy
production.'
Davies
et
al.
(1967)
went
a
step
further,
in
suggesting
the
nature
of
the
postulated
reversible
process.
Using
an
experimental
design
especially
pro-
posed
by
Hill
(1966),
they
did
not
find
any
chemical
equivalent
of
the
shortening
heat
in
single
tetanic
contraction
with
free
shortening,
inter-
rupted
by
freezing
before
relaxation.
According
to
them,
'the
heat
of
short-
ening
is
most
certainly
not
degraded
free
energy
from
the
splitting
of
ATP,
and
must
therefore
be
transformed
entropy
from
some
other
(cyclic)
process'.
The
conclusions
of
both
Carlson
et
al.
(1963)
and
Davies
et
al.
(1967)
are
clearly
contradictory
to
Fenn's
result,
and
it
was
thus
important
to
check
the
existence
of
a
net
thermal
effect
of
the
shortening,
in
conditions
allowing
the
best
comparison
with
isometric
contractions.
A
preliminary
report
of
this
work
has
been
published
(Lebacq
&
Aubert,
1970).
182

HEAT
OF
SHORTENING
IN
MUSCLE
183
METHODS
The
muscle8
The
experiments
were
made
on
sartorius
muscles
of
male
Rana
temporaria
at
00
C.
Eleven
pairs
of
muscles
have
been
used.
The
average
weight
of
a
pair
was
193
mg.
After
dissection,
the
muscles
were
mounted
on
the
thermopile
at
the
standard
length
lo
and
placed
in
a
chamber
filled
with
Ringer
solution
containing
NaCl
116
mm,
KCl
2
mm,
MgCl2
1
mm,
phosphate
buffer
(adjusted
to
pH
7-32)
2
mm,
where
they
were
kept
for
3
hr
before
stimulation.
The
muscle
chamber
was
immersed
in
a
Dewar
flask
containing
a
mixture
of
ice
and
water,
continuously
stirred
by
air
blowing.
Force
meawurement8
The
pair
of
muscles
was
tied
to
a
light
silver
chain
by
two
small
cotton
threads
fastened
together
by
a
strong
knot.
The
cotton
link
used
was
as
short
as
possible,
about
1
mm
in
length.
The
chain
was
suspended
to
strain
gauges
fixed
to
the
arm
of
an
ergometer;
the
tension
was
recorded
on
one
beam
of
a
dual-beam
oscilloscope
(Aubert,
1956,
p.
53).
Heat
measurements
We
have
used
a
thermopile
made
of
two
sets
of
18
constantan-nichrome
couples,
which
could
be
used
either
separately
or
connected
in
series
or
in
parallel
(see
Downing's
D
1;
Abbott,
Aubert
&
Hill,
1952).
Each
set
of
couples
gives
760
1sV/0
C
at
00
C
(calibration
made
on
a
single
couple
and
checked
on
each
set
of
couples
separately)
and
is
9
mm
long.
The
total
length
of
the
pile
is
25
mm,
including
three
stimulating
electrodes
and
a
few
protecting
couples.
The
heat
signal
was
amplified
photo-electrically
and
recorded
either
on
the
second
beam
of
the
oscilloscope
or
on
an
X-Y
recorder
(Moseley
2D2,
Pasadena)
with
a
25
cm
maximum
deflexion.
Correction
for
heat
losses
was
usually
worked
out
by
calculation,
though
in
several
experiments
we
have
used
an
automatic
electronic
correction.
To
facilitate
the
grouping
of
results
from
muscles
of
different
size,
it
was
found
convenient
to
divide
the
heat
value
(given
in
g.
cm)
by
the
product
of
P.
(isometric
tetanic
tension
at
reference
length
in
g-force)
and
1.
(standard
length
in
cm).
Stimulation
and
movement
Condenser
discharges
of
alternated
polarity
were
applied
through
three
stimulating
electrodes
built
in
the
thermopile,
one
in
the
middle,
and
two
connected
together
at
both
edges
of
the
pile.
The
frequency
of
stimulation
was
20/sec;
the
duration
of
the
train
of
discharges
was
either
1-2
or
2
sec.
The
shortening
was
effected
at
constant
velocity,
under
the
control
of
a
Levin-
Wyman
ergometer.
The
movement
was
normally
triggered
after
0-4
sec
of
stimula-
tion,
at
the
standard
length
I.,
at
a
time
when
tension
had
nearly
reached
a
steady
level.
It
was
restricted
to
4-27
mm,
and
was
performed
in
0-2
sec.
This
high
velocity
was
chosen
so
as to
show
clearly
the
shortening
heat
and
minimize
the
correction
of
the
length
change
in
the
isometric
reference
tetanus
(Hill,
1938).
After
the
end
of
the
movement,
the
stimulation
continued
long
enough
in
order
that
the
tension
reached
a
new
steady
level
at
the
shorter
length.
In
three
sets
of
experiments
a
longer
duration
of
stimulation
was
used
(2
sec),
so
as
to
compare
the
effects
of
an
early
(from
0-4
to
0-6
sec)
and
a
late
shortening
(from
1-2
to
1-4
see).

X.
AUBERT
AND
J.
LEBACQ
Experimental
design
The
minimum
set
of
contractions
consisted
either
of
three
or
of
four
tetanic
con-
tractions
(isometric
at
the
two
extreme
lengths
and
tetani
with
shortening
at
0 4
see
or
at
04
and
12
sec).
After
thermal
equilibration
in
moistened
oxygen,
the
muscle
received
the
series
of
three
or
four
different
stimulations
at
15
min
intervals.
It
was
then
rinsed
for
10
min
in
oxygenated
Ringer
solution
and
the
series
was
repeated
in
a
different
order.
In
most
cases
a
simple
mirror
design
was
adopted
(series
and
reverse),
sometimes
repeated
twice.
In
every
case,
the
results
are
given
as
the
average
curves
of
tension
or
heat
production
for
the
three
or
four
different
experimental
conditions
and
are
thus
largely
independent
of
the
possible
influence
of
fatigue.
In
the
batch
of
frogs
used,
however,
the
tension
remained
in
most
cases
constant
within
1
%
from
beginning
to
end
of
an
experiment,
including
sometimes
sixteen
tetani.
Except
when
it
is
explicitly
mentioned,
the
results
are
given
for
the
two
halves
of
the
thermopile
taken
together,
so
as
to
get
the
best
estimate
of
the
heat
produced
by
the
whole
muscle.
1.
The
tension
curves
RESULTS
Fig.
1
shows
the
tension
developed
in
the
three
conditions
of
an
experi-
ment
with
early
release
during
a
2
see
tetanus.
A
is
the
isometric
at
the
initial
length
(isometric
'long');
B
is
the
isometric
at
the
final
length
(isometric
'short');
C
is
the
contraction
with
shortening
starting
after
04
see
of
isometric
'long'.
It
is
seen
that,
as
a
result
of
the
high
velocity
of
shortening
(2.14
cm/sec),
the
tension
(P)
falls
appreciably
during
the
shortening
(P/IPO
0
020).
After
the
end
of
the
shortening,
tension
rises
again
and
reaches
an
isometric
steady
level
at
1
sec,
which
remains
constant
for
the
next
second
of
stimulation.
Table
1
shows
the
tension
at
the
time
of
the
last
stimulus:
this
was
lower
than
the
tension
developed
at
the
same
length
during
a
tetanus
isometric
throughout
(i.e.
without
release
from
a
larger
length).
This
is
true
whether
the
isometric
tension
is
larger
or
smaller
at
the
initial
length
than
at
the
final
length.
It
is
the
case
for
instance
in
experiments
Nos.
1
and
9:
after
shortening,
the
tension
rises
above
the
level
of
the
isometric
'long'
but
remains
lower
than
the
level
corresponding
to
the
isometric
'short'.
This
confirms
earlier
observations
of
Abbott
&
Aubert
(1952)
on
dogfish
jaw
muscle
and
of
Aubert
(1956)
on
frog
sartorius.
In
order
to
test
the
influence
of
the
post-shortening
period
of
activity
on
the
recovery
of
tension
as
well
as
on
the
evolution
of
the
shortening
heat,
in
three
experiments
with
2
see
tetani,
the
release
was
triggered
at
04
see
(early
shortening)
or
at
1-2
see
(late
shortening)
(Expts.
4,
5
and
6
in
Table
1).
In
each
case
the
isometric
tension
redeveloped
after
shortening
was
somewhat
lower
after
a
late
shortening
than
after
an
early
shortening.
It
should
be
emphasized
that
in
the
experiments
of
Table
1,
stimulation
was
continued
long
enough
to
allow
the
tension
to
reach
a
steady
level
after
the
movement
was
over.
184

HEAT
OF
SHORTENING
IN
MUSCLE
2.
The
total
heat
produced
during
contraction
and
relaxation
Fig.
2
shows
the
average
recordings
of
the
heat
produced
during
an
experiment
with
shortening
starting
either
at
04
or
at
1
2
see
during
iso-
metric
tetani
of
2
see
duration.
In
this
example,
the
isometric
'long'
and
'short'
(A
and
B)
are
practically
the
same
during
the
contraction
period
and
diverge
only
during
relaxation.
As
the
shortened
muscle
relaxes
in
the
'short'
condition,
it
is
appropriate
to
compare
the
curves
C
and
C',
427
mm
Length
L
.lLL..L~L.LL
LJX..
.U..
.
".
4|
sIJ
Stimuli
0
100
05
1-0
20
2-5
30
Time
(sec)
Fig.
1.
Time
course
of
tension
in
2
sec
tetani.
A:
isometric
at
longer
length;
B:
isometric
at
shorter
length;
C:
tetanus
with
shortening;
inter-
rupted
line
D:
the
reference
tetanus
corrected
for
the
length
change.
In
the
top
left
corner,
the
length
of
the
muscle
in
tetanus
C
and
the
train
of
stimuli.
Vertical
bars:
04
sec
blocks
as
used
in
Section
3.
[Note:
time
scale
interrupted
between
1-1
and
1-9
sec
(Expt.
4:
P.:
177-7
g-force;
1:3-0
cm;
muscle
weight:
0-227
g).]
referring
respectively
to
the
early
and
late
shortening,
with
curve
B.
The
differences
between
these
curves
are
shown
on an
enlarged
scale
in
curves
D
and
D'.
It
can
be
remarked
first
that
the
supplement
of
heat
produced
as
a
result
of
the
shortening
is
hardly
different
for
the
early
and
the
late
shortening.
Secondly,
the
extra
heat
has
not
vanished
at
the
end
of
relaxa-
tion:
it
slightly
increases
immediately
after
the
end
of
the
movement,
remains
constant
up
to
the
end
of
the
stimulation
period
and
during
the
first
0*6
sec
of
relaxation;
then
it
decreases,
by
about
20
%
in
this
case,
during
the
last
stage
of
relaxation.
185

186
X.
AUBERT
AND
J.
LEBACQ
As
the
main
problem
concerns
the
fate
of
the
shortening
heat
during
relaxation,
the
crudest
analysis
of
the
results
should
at
least
separate
the
heat
produced
during
the
contraction
and
the
relaxation
periods.
As
a
first
indication
of
the
general
trend,
Table
2
gives
first
the
average
heat
produced
in
each
condition
up
to
the
last
stimulus
(column
labelled
'
contraction');
then
the
heat
produced
during
1F6
sec
after
the
last
shock,
i.e.
up
to
the
complete
disappearance
of
tension
(column
'relaxation');
and
finally
the
sum
of
both
(column
'total').
The
analysis
is
given
separ-
ately
for
the
1F2
see
tetani
(n
=
8),
with
early
release,
and
for
the
2
sec
tetani
(n
=
3),
where
both
early
and
late
shortening
have
been
applied
to
the
same
muscles.
TABLE
1.
The
muscle
tension
at
the
time
of
the
last
stimulus:
isometric
tetani
at
both
initial
('long')
and
final
('short')
length
and
tetani
with
shortening.
In
Expts.
4,
5
and
6
the
stimulation
lasted
2
sec.
In
all
other
experiments
the
duration
was
1-2
sec.
Shortening
was
between
0
4
and
0-6
sec
in
all
but
the
three
'late'
shortenings
(Expts.
4,
5
and
6)
when
it
occurred
between
1-2
and
1-4
sec
Tension
(g-force)
Isometric
Muscle
Muscle
Isometric
'short'
Tetani
with
shortening
length
weight
'long'
(l
(from
lo
to
-
4-27
mm)
Expt.
4o
(cm)
(mg)
(lo)
-4o27
mm)
early
late
1
2-95
167
70-5
80.4
72-5
2
3-10
230
140*8
124.6
119*5
-
3
3.30
348
181-0
167.0
156.4
-
4
300
227
177-7
150-0
143.0
139.0
5
2-95
215
144.5
139*1
134-2
131.5
6
305
131
86-0
80-3
77*0
76-4
7
2.90
177
128.6
115*2
108.1
8
2-85
168
133-5
133-3
126-1
9
2-75
137
98-6
110-7
103-7
10
2-90
155
117.0
113
6
108-4
11
2.80
168
132-2
133-7
124-6
All
the
heat
values
have
been
expressed
in
terms
of
the
product
P.
lo
to
normalize
the
results
(see
Methods).
As
a
first
approximation
of
the
effect
of
shortening,
the
heat
values
for
the
contraction
with
shortening
may
be
compared
with
the
'isometric
short',
as
in
curves
D
and
D'
of
Fig.
2.
How-
ever,
to
assess
the
significance
of
these
differences,
one
has
to
take
into
consideration
the
planning
of
the
experiments,
which
reduces
their
standard
errors.
This
has
been
done
in
Table
2.
The
differences
are
highly
significant
for
the
total
duration
of
the
con-
traction-relaxation
period
as
well
as
for
the
contraction
period.
Thus
it
is
quite
obvious
that
a
muscle
shortened
during
contraction
produces
an

HEAT
OF
SHORTENING
IN
MUSCLE
187
excess
of
heat,
that
is
not
compensated
by
an
equal
heat
absorption
during
relaxation.
Nevertheless
the
excess
is
reduced
by
15-25
%
during
that
period.
This
might
be
related
with
the
smaller
tension
of
the
shortened
muscle
at
the
time
of
relaxation
(see
5).
3.
Analysis
of
the
heat
production
in
blocks
of
04
sec
The
results
given
in
the
preceding
section
are
adequate
for
com-
parisons
with
the
results
obtained
by
biochemical
techniques.
But
the
150
t°
100
I00
45
50
E
20
-4
I
10
1
2
3
Time
(sec)
Fig.
2.
Cumulated
heat
production
in
2
sec
tetani.
A:
isometric
'long';
B:
isometric
'short';
C:
tetanus
with
early
shortening;
C':
tetanus
with
late
shortening;
D
=
C
-
B
and
D'
=
C'-
B
on
enlarged
scale
(at
the
right
of
the
Figure).
Upper
traces:
stimulation
period
and
muscle
length
during
tetani
C
and
C'
(Expt.
5:
PF:
144*5
g-force;
lo:
2-95
cm;
muscle
weight:
0215
g.
Means
of
four
traces).

X.
AUBERT
AND
J.
LEBACQ
myothermic
method
allows
a
finer
analysis
of
the
effects
of
the
shortening
at
any
time
during
the
contraction-relaxation
cycle
(see
Fig.
2).
For
our
purpose
it
was
accurate
enough
to
make
the
analysis
in
blocks
of
0-4
sec.
The
choice
of
this
interval
is
justified
because
it
includes
in
the
same
period
the
time
required
for
the
shortening
(0-2
see)
and
for
most
of
TABLE
2.
Total
heat
production
during
contraction
and
relaxation,
in
three
experi-
mental
conditions:
isometric
tetani
at
the
longer
length
(lo)
and
at
the
shorter
length
(lo-
427
mm);
tetani
with
shortening
(from
lo
to
lo
-
4-27
mm).
Upper
part
of
table:
1-2
sec
stimulation;
release
0
4
sec
after
first
stimulus.
Lower
part
of
table:
2-0
sec
stimulation;
release
either
0-4
sec
(early
shortening)
or
1
2
sec
(late
shorten-
ing)
after
first
stimulus.
All
values
of
heat
(
S.E.
of
mean)
are
given
in
dimensionless
units
(g.
cm
of
heat
x
1000/PO
lo)
1-2
sec
tetani
(n
=
8;
average
P.:
125
g-force;
average
lo:
2-9
cm;
average
muscle
weight:
04194
g)
Contraction
Relaxation
Total
Isometric
'long'
183-5
+
6-9
86-1
+
6-5
269-6
+
9-7
Isometric
'short'
181-0
+
9.7
77-8
+58
25*8+
12-5
Shortening
215-0
+
9-9
73-3
+46
288'3+
11.1
Shortening
minus
isometric
34
0
+
3X3
-4.5
+
2-7
29*5
+
5*6
'short'
(P
<
0.001)
(P
<
0.01)
2-0
sec
tetani
(n
=
3;
average
P.:
136
g-force;
average
lo:
3-0
cm;
average
muscle
weight:
0-191
g)
Contraction
Relaxation
Total
Isometric
'long'
301-0+
14-8
98-3
+
12-3
399.3
+
26-0
Isometric
'short'
296-3
+
14-7
77-3
+
2-6
373-7
+
16-7
Early
shortening
334-0
+
15-5
71-3
+
2-2
405
3
±
14-8
Late
shortening
338-3
+
16-2
67-3
±
3.8
405
7
+
15-5
Early
shortening
minu8
37.7
+
1-2
-
60
+
3-1
31-7
+
2-6
isometric
'short'
(Pa
0.001)
(P
<
0.01)
Late
shortening
minus
42-0
+
1-7
-10
0
+
3-8
32-0
+
2-1
isometric
'short'
(P
<
0.01)
(P
<
0.01)
the
subsequent
redevelopment
of
tension
(up
to
90-95
%
of
the
final
isometric
level:
see
Fig.
1).
This
largely
avoids
the
necessity
of
correction
for
an
eventual
thermoelastic
effect
associated
with
the
change
of
tension
(Woledge,
1961).
Table
3
gives
an
example
of
this
procedure.
The
instantaneous
effect
of
shortening
is
obtained
by
comparing
the
corresponding
heat
rate
(line
4
of
the
Table)
with
an
artificial
isometric
reference,
taking
the
change
of
length
into
account
(line
5).
Though
there
is
something
arbitrary
in
the
procedure
(see
Aubert,
1956,
pp.
245
ff.;
Mommaerts,
1970),
it
seems
reasonable
to
take
as
reference
before
the
movement
starts
the
heat
rate
at
the
greater
length
(line
2),
and
from
the
end
of
the
movement
onwards
the
heat
rate
at
the
shorter
length
(line
3).
During
the
period
occupied
by
the
shortening
and
the
redevelopment
of
tension,
we
have
assumed
that
the
heat
rate
was
'long'
for
0-1
sec
and
'short'
for
0
3
sec,
since
the
complete
shortening
itself
took
place
during
the
first
half
of
the
period
considered.
In
most
cases
the
heat
rates
are
hardly
different
for
'long'
and
'short',
188

HEAT
OF
SHORTENING
IN
MUSCLE
EH
H
o
sXe
e
B
:
C5
>
o
s
00
o
o
o
1
co
=
:sl
CA
c
1010
CB
4S
CD
0t
CO
Ci
*
m
I"
'5D
Noo
o
cs
:Or:
I-
*
.
L-
0
r-
X
1-
1o
COC6
5~
.s
E
O~
S
*
*
*
*
*
189
0
C
O
.)
Eo
4
0c
C;
4-0
0
_
0
0
r0
,2,
o>
e
o
.P
E-q
*0
C)
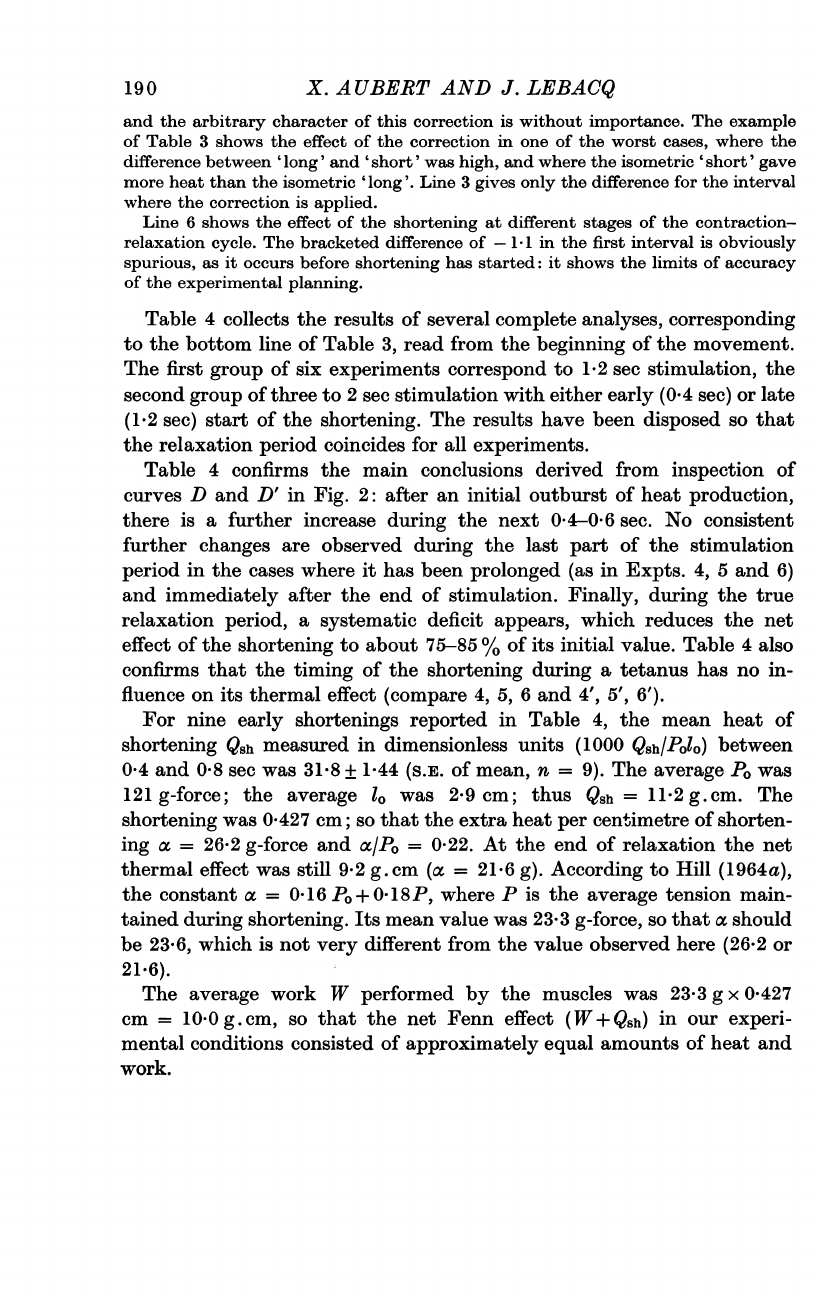
X.
A
UBERT
AND
J.
LEBACQ
and
the
arbitrary
character
of
this
correction
is
without
importance.
The
example
of
Table
3
shows
the
effect
of
the
correction
in
one
of
the
worst
cases,
where
the
difference
between
'long'
and
'short'
was
high,
and
where
the
isometric
'short'
gave
more
heat
than
the
isometric
'long'.
Line
3
gives
only
the
difference
for
the
interval
where
the
correction
is
applied.
Line
6
shows
the
effect
of
the
shortening
at
different
stages
of
the
contraction-
relaxation
cycle.
The
bracketed
difference
of
-
11
in
the
first
interval
is
obviously
spurious,
as
it
occurs
before
shortening
has
started:
it
shows
the
limits
of
accuracy
of
the
experimental
planning.
Table
4
collects
the
results
of
several
complete
analyses,
corresponding
to
the
bottom
line
of
Table
3,
read
from
the
beginning
of
the
movement.
The
first
group
of
six
experiments
correspond
to
1-2
see
stimulation,
the
second
group
of
three
to
2
sec
stimulation
with
either
early
(0.4
see)
or
late
(1.2
sec)
start
of
the
shortening.
The
results
have
been
disposed
so
that
the
relaxation
period
coincides
for
all
experiments.
Table
4
confirms
the
main
conclusions
derived
from
inspection
of
curves
D
and
D'
in
Fig.
2:
after
an
initial
outburst
of
heat
production,
there
is
a
further
increase
during
the
next
0-4-0-6
sec.
No
consistent
further
changes
are
observed
during
the
last
part
of
the
stimulation
period
in
the
cases
where
it
has
been
prolonged
(as
in
Expts.
4,
5
and
6)
and
immediately
after
the
end
of
stimulation.
Finally,
during
the
true
relaxation
period,
a
systematic
deficit
appears,
which
reduces
the
net
effect
of
the
shortening
to
about
75-85
%
of
its
initial
value.
Table
4
also
confirms
that
the
timing
of
the
shortening
during
a
tetanus
has
no
in-
fluence
on
its
thermal
effect
(compare
4,
5,
6
and
4',
5',
6').
For
nine
early
shortenings
reported
in
Table
4,
the
mean
heat
of
shortening
Qsh
measured
in
dimensionless
units
(1000
Qsh/Polo)
between
0
4
and
0-8
sec
was
318
+
1*44
(s.E.
of
mean,
n
=
9).
The
average
PO
was
121
g-force;
the
average
lo
was
2*9
cm;
thus
Qsh
=
11
2
g.cm.
The
shortening
was
0-427
cm;
so
that
the
extra
heat
per
centimetre
of
shorten-
ing
a
=
26*2
g-force
and
t/PO
=
0-22.
At
the
end
of
relaxation
the
net
thermal
effect
was
still
9
2
g.
cm
(ax
=
21*6
g).
According
to
Hill
(1964a),
the
constant
ax
=
0
16
PO
+
0.18P,
where
P
is
the
average
tension
main-
tained
during
shortening.
Its
mean
value
was
23-3
g-force,
so
that
a
should
be
23-6,
which
is
not
very
different
from
the
value
observed
here
(26.2
or
21.6).
The
average
work
W
performed
by
the
muscles
was
23-3
g
x
0
427
cm
=
10.0g.cm,
so
that
the
net
Fenn
effect
(W+Qsh)
in
our
experi-
mental
conditions
consisted
of
approximately
equal
amounts
of
heat
and
work.
190

HEAT
OF
SHORTENING
IN
MUSCLE
0
EH
0
H
rO
1CO
o
o-o0q
c-
*
=
C*
aq
m
to
UZ
m
=
O0
C=
O1
to
=
_-
C;
_:
o
cq
cw
,<
I
I
I
rt-
"-
aq
InC
eq
t
_1
e1
0
qc
E--q
*"
1
4*
.
q
*
I
I"
0
11
=
01X0
0
O
O
CO
CO
0
10
.4
0
0)
01
aq
O0
t-
10
cq
C*u
COCOCO
C
C
COCO
00
0~~~0"
OO0
O
Oq
14
4c
ac
00S
CO00
0
O.
*
*
m
q
SCO
I*
*
1
1
oo
00
o
cqI
-I
0
cO
lCO
C
*
*
m
aq
00
6
-
.B
-
CO C
to
.5
0
1.4
0
w
14
co
ci
0
01
bo
.5
0
&4
PHY
216
191
o
5
4
0
Q
ra-e
(:a
0
(D
E-4
*45
CO
0
0
o
*g
.z
o
o
w0
0
bo
;
4
o
.A
O
t-4
4
W
0
°-
A0
(D
C)
°
O
7
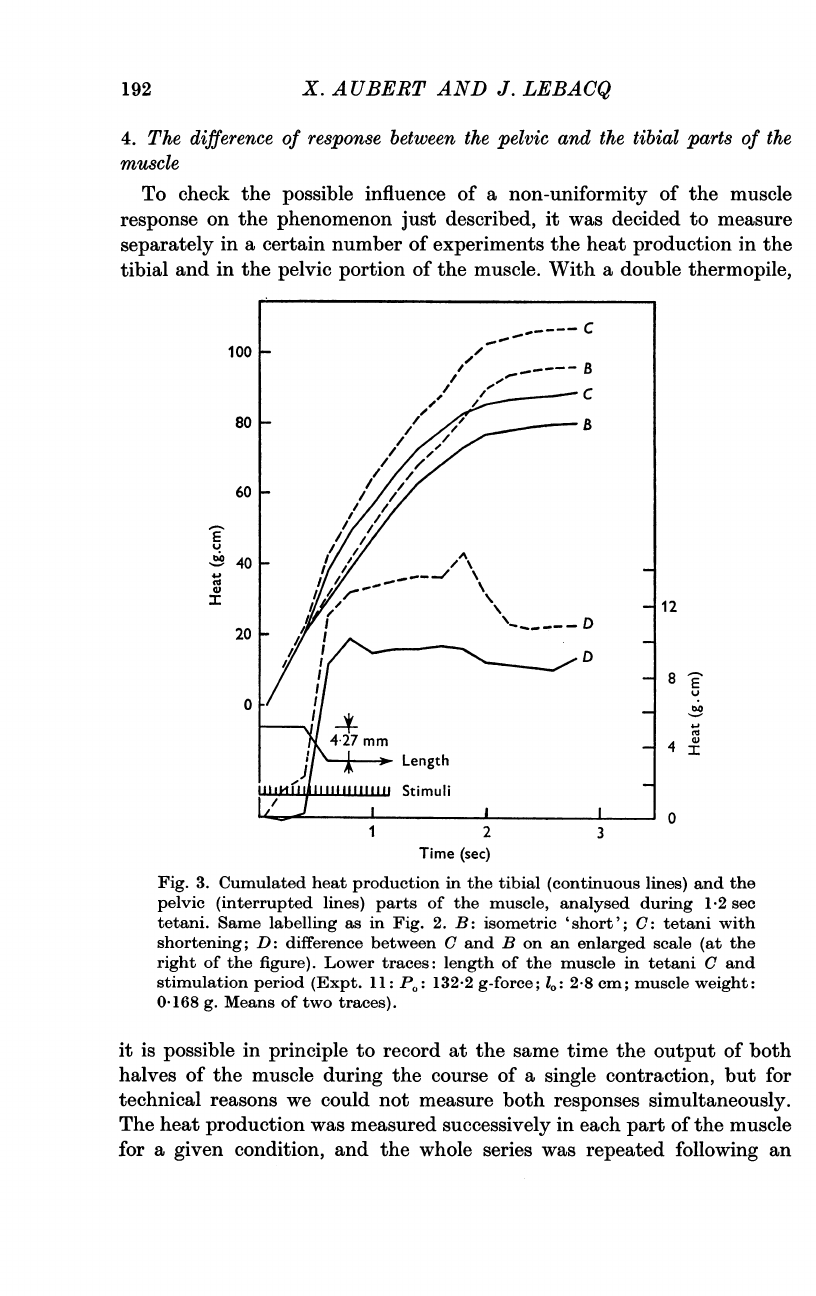
X.
AUBERT
AND
J.
LEBACQ
4.
The
difference
of
response
between
the
pelvic
and
the
tibial
parts
of
the
muscle
To
check
the
possible
influence
of
a
non-uniformity
of
the
muscle
response
on
the
phenomenon
just
described,
it
was
decided
to
measure
separately
in
a
certain
number
of
experiments
the
heat
production
in
the
tibial
and
in
the
pelvic
portion
of
the
muscle.
With
a
double
thermopile,
100
80
60
-"
40
Cd
I
20
0
1
2
3
Time
(sec)
12
OE
4T_
o
Fig.
3.
Cumulated
heat
production
in
the
tibial
(continuous
lines)
and
the
pelvic
(interrupted
lines)
parts
of
the
muscle,
analysed
during
1
2
sec
tetani.
Same
labelling
as
in
Fig.
2.
B:
isometric
'short';
C:
tetani
with
shortening;
D:
difference
between
C
and
B
on
an
enlarged
scale
(at
the
right
of
the
figure).
Lower
traces:
length of
the
muscle
in
tetani
C
and
stimulation
period
(Expt.
11:
PF:
132-2
g-force;
lo:
2-8
cm;
muscle
weight:
0-168
g.
Means
of
two
traces).
it
is
possible
in
principle
to
record
at
the
same
time
the
output
of
both
halves
of
the
muscle
during
the
course
of
a
single
contraction,
but
for
technical
reasons
we
could
not
measure
both
responses
simultaneously.
The
heat
production
was
measured
successively
in
each
part
of
the
muscle
for
a
given
condition,
and
the
whole
series
was
repeated
following
an
192

HEAT
OF
SHORTENING
IN
MUSCLE
adequate
experimental
design.
As
the
muscles
were
in
excellent
condition
this
procedure
hardly
affected
the
accuracy
of
the
comparisons
made
on
the
average
curves.
The
differences
observed
are
illustrated
for
a
typical
experiment
in
Fig.
3,
which
has
the
same
disposition
as
Fig.
2,
except
that
for
clarity
the
curves
corresponding
to
isometric
'long'
have
been
omitted
(curve
A
in
Fig.
2).
The
curves
in
full
lines
correspond
to
the
tibial
part
of
the
muscle
TABLE
5.
Comparison
of
the
heat
production
in
the
pelvic
and
in
the
tibial
portion
of
muscle
during
contraction
and
relaxation.
All
values
of
heat
(
S.E.
of
mean)
are
given
in
dimensionless
units
(g.
cm
of
heat
x
1000/Po
1o)
1.
Pelvic
heat
minus
tibial
heat,
in
the
three
experimental
conditions
(n
=
4)
Contraction
Relaxation
Total
Isometric
'long'
16-5
+
6-0
50-8
+
8-5
67.3
+
13-9
Isometric
'short'
6-0
+38
273
+6*3
33-3
±
9.1
Tetanus
with
shortening
8-0+
12.1
11-5
+
9
0
19-5
+
17.2
2.
Effect
of
shortening
(corrected
for
length
changes
as
indicated
in
Table
3)
in
the
pelvic
and
in
the
tibial
portion
of
muscle.
In
brackets,
results
of
one
experiment
with
the
muscles
reversed
upside
down
on
the
thermopile
(see
explanation
in
text)
(n
=
8)
Contraction
Relaxation
Total
Tibial
37-5±341
1-5+3-3
39
0+4
0
(38.2)
(1.4)
(39-6)
Pelvic
33-2
+
2-3
-12-3
+
3-4
20-9
+
2-5
(30-9)
(-19-7)
(11.2)
and
those
in
interrupted
line
to
the
pelvic
part;
B
is
the
isometric
'short'
tetanus,
C
the
tetanus
with
shortening
and
D
the
difference
between
B
and
C
on
an
enlarged
scale.
Three
main
differences
are
noticeable:
heat
production
is
larger
in
the
pelvic
than
in
the
tibial
portion
of
the
muscle;
during
relaxation,
the
outburst
of
heat
is
much
more
conspicuous
in
the
pelvic
part
(the
difference
was
still
more
marked
for
the
isometric
'long'
tetanus
not
shown
in
the
figure);
finally
the
decrease
during
relaxation
of
the
excess
of
heat
due
to
the
shortening
is
about
25
%
in
the
pelvic
portion
but
only
12
%
in
the
tibial
portion.
Table
5
confirms
these
general
results.
In
the
upper
part
are
shown
the
average
differences
between
the
pelvic
and
the
tibial
parts
for
five
such
differential
experiments
with
1-2
see
tetani,
analysed
separately
during
contraction
and
relaxation,
as
in
Table
2.
These
differences
should
be
compared
with
the
absolute
values
given
in
the
upper
part
of
Table
2.
The
lower
part
of
Table
5
compares
for
both
halves
of
the
thermopile
the
average
effect
of
the
shortening
corrected
for
the
change
of
length
as
explained
in
Table
3.
In
this
part
of
Table
5,
the
results
for
both
types
of
7-2
193

X.
AUBERT
AND
J.
LEBACQ
shortening
(early
or
late)
are
included
whatever
the
duration
of
the
stimulation
(1.2
or
2-0
sec),
as
these
factors
proved
to
have
no
influence
on
the
effect
of
shortening
(see
also
Table
4).
It
appears
that
the
effect
of
shortening
is
more
marked
in
the
tibial
part
of
the
muscle.
Hardly
significant
during
contraction,
the
difference
between
the
two
parts
becomes
obvious
for
the
total
contraction-relaxa-
tion
cycle,
because
of
the
larger
and
significant
decrease
of
heat
produc-
tion
in
the
pelvic
part
during
relaxation.
If
the
lower
half
of
the
thermopile
correctly
estimates
the
heat
production
in
the
pelvic
portion
of
the
muscle,
it
must
be
admitted
that the
upper
part
samples
only
a
portion
of
the
tibial
end
of
the
muscle.
Therefore
one
might
wonder
whether
the
portion
of
the
muscle
above
the
top
edge
of
the
thermopile
might
not
exhibit
a
still
more
substantial
reduction
which,
together
with
the
effect
at
the
lower
end,
could
completely
cancel
the
shortening
heat
as
measured
in
the
main
portion
of
the
muscle.
This
is
most
unlikely,
because
the
extreme
tibial
part
of
the
muscle
not
lying
on
the
thermopile
represents
only
a
small
fraction
of
the
muscle's
weight,
especially
at
the
shorter
length
where
relaxation
occurs.
Besides,
in
one
experiment
a
pair
of
muscles
was
mounted
upside
down
on
the
thermopile,
so
that
the
whole
tibial
end
of
the
muscle
was
in
contact
with
the
lower
half
of
the
pile.
As
shown
by
the
values
in
brackets
in
the
lower
part
of
Table
5,
that
portion
behaved
exactly
like
the
middle
part
of
the
muscle,
in
showing
no
reduction
of
the
heat
of
shortening.
5.
The
correlation
between
relaxation
heat
and
the
final
tension
As
shown
in
the
first
section
(Table
1
and
Fig.
1),
the
tension
redeveloped
after
an
active
shortening
is
always
lower
at
the
beginning
of
relaxation
than
the
corresponding
isometric
tension
at
the
same
length.
This
pheno-
menon
could
help
to
explain
the
deficit
of
heat
observed
during
relaxation
after
a
shortening.
According
to
Hill
(1961),
three
main
factors
account
for
the
heat
produced
after
the
last
stimulus:
(1)
the
mechanical
energy
stored
in
the
series
elastic
elements
is
probably
dissipated
as
heat
during
relaxation;
(2)
the
fall
of
tension
must
be
associated
with
a
reversible
thermoelastic
effect;
and
(3)
some
residual
activity
must
be
expected
(even
in
a
twitch
the
active
state
does
not
disappear
instantaneously).
All
three
factors
depend
somehow
on
the
final
tension.
It
might
be
possible
to
attempt
a
quantitative
analysis
of
their
relative
importance.
But
it
is
more
instruc-
tive
to
examine
the
general
relation
between
the
tension
at
the
time
of
the
last
stimulus
and
the
heat
produced
during
relaxation.
Fig.
4
shows
this
relation
for
the
three
different
conditions
of
each
individual
experiment.
The
correlation
between
relaxation
heat
and
tension
is
obvious
and,
what
is
more
important,
no
systematic
difference
appears
to
exist
between
the
three
types
of
contraction:
on
the
basis
of
this
empirical
diagram
it
is
impossible
to
discern
a
contraction
with
shortening
from
an
isometric
contraction.
194

HEAT
OF
SHORTENING
IN
MUSCLE
195
.3
.4
60
X3
40
~~~~~~~~~~~80
2004
03
40
2*
*s°
°
80
0X
0
8x
%4
ba
010
~~~
~~~~~~~11
oil
4.1
lox0
x27
0
o10
x9
07
20
06
o2
X
.06
l9
ol
.1
80
100
140
180
Tension
at
time
of
last
stimulus
(g)
Fig.
4.
Total
heat
produced
after
the
last
stimulus
plotted
against
the
tension
at
the
time
of
the
last
stimulus.
Double
logarithmic
scales.
Filled
circles:
isometric
'long';
open
circles:
isometric
'short';
crosses:
tetani
with
shortening.
The
figures
beside
the
symbols
refer
to
the
Expt.
number
as
given
in
Table
1.
DISCUSSION
1.
Does
shortening
increase
the
net
heat
production
of
muscle?
The
very
existence
of
a
net
effect
of
shortening
on
the
heat
production
of
muscle
has
been
questioned.
Trying
to
reconcile
the
apparent
contra-
diction
between
the
results
of
Carlson
et
al.
(1963)
and
Hill
(1938,
1949,
1964c),
Kushmerick
et
al.
(1969)
suggested
that:
'shortening
heat
must
be
part
of
a
cyclic
process,
since
it
is
not
seen
in
the
whole
of
the
contraction-
relaxation
cycle,
whereas
it
is
while
the
shortening
proceeds'.
The
purpose
of
this
paper
was
to
check
this
point,
and
the
experimental
conditions
have
been
carefully
chosen to
serve
this
purpose.
(1)
The
shortening
always
occurred
during
a
steady
state
of
tetanic
activity,
rather
than
in
the
course
of
twitches,
to
avoid
the
possible
influence
of
the
release
on
this
course,
as
a
result
of
change
of
'active
state'
or
of
positive
feed-back
effects
of
the
tension
(Pringle,
1960;
Hill,
1964b).
(2)
The
movement
was
controlled
at
constant
velocity
with
an
ergometer,
rather
than
at
constant
load
through
an
isotonic
lever,
in
order
to
collect
the
mechanical
work
externally
and
to
avoid
its
dissipation
as
heat
in
the
muscle
by
the
falling
load
during

X.
AUBERT
AND
J.
LEBACQ
relaxation.
(3)
The
choice
of
a
rapid
speed
of
shortening
simplified
the
comparison
with
the
isometric
contractions
for,
except
during
the
short
period
of
the
movement,
the
muscle
remained
at
constant
length,
either
long
or
short,
during
the
main
part
of
the
contraction-relaxation
cycle
(see
Fig.
1).
(4)
The
extent
of
the
shortening
was
limited
(4.27
mm)
so
that
the
isometric
heat
production
at
both
lengths
was
not
too
different.
The
results
collected
in
Tables
2
and
4,
and
illustrated
in
Fig.
2,
demon-
strate
clearly
that
in
those
experimental
conditions
shortening
induces
an
appreciable
thermal
effect,
in
the
form
of
a
supplement
to
the
iso-
metric
heat,
measurable
at
the
end
of
relaxation
as
well
as
during
the
TABLE
6.
Possible
evidence
for
shortening
heat
in
the
case
of
a
series
of
isotonic
contractions
(calculated
from
Fales,
1969).
Each
value
of
total
energy
output
and
of
total
work
is
the
sum
of
the
results
obtained
for
a
given
load
P
from
eight
pairs
of
muscle
performing
a
series
of
five
after-loaded
isotonic
twitches.
The
load
is
given
as
the
average
ratio
P/PO
of
the
eight
muscles.
In
Fales'
experiments,
the
different
isotonic
series
were
performed
in
a
randomized
order
between
two
isometric
series.
The
isometric
data
are
given
in
the
two
columns
labelled
100.
All
values
of
energy
and
work
are
in
ergs
x
104/g
of
muscle
100
P/P0
100
64
43
29
16
6
100
Total
initial
energy
(i.e.
total
heat
2010
2216
3010
3232
2984
2114
1764
recorded)
Total
work
0
232
717
710
573
243
0
Heat
produced
by
the
muscle
2010
1984
2293
2522
2411
1871
1764
(initial
energy
minws
work)
Isotonic
heat
minus
mean
-
97
406
635
524
-16
-
isometric
heat
movement
itself.
The
general
propositions
of
Carlson
et
al.
(1963)
and
of
Kushmerick
et
al.
(1969)
are
thus
not
verified:
the
heat
of
shortening
is
not
reversed
during
relaxation;
it
must
imply
the
dissipation
of
free
energy.
The
same
conclusions
can
be
deduced
from
recent
works
by
Fales
(1969)
and
by
Jdbsis
&
Duffield
(1967).
Using
gradient-layer
calorimetry,
Fales
measured
the
total
energy
produced
by
frog
sartorius
muscle
per-
forming
a
series
of
five
short
tetanic
contractions
under
isometric
and
different
isotonic
conditions
(as
in
Fenn's
original
experiments,
the
work
produced
during
shortening
was
dissipated
in
the
muscle
as
heat
by
the
load
falling
during
relaxation).
In
his
table
1,
Fales
gives
the
values
of
work
and
total
energy
of
each
individual
case,
from
which
it
is
possible
to
calculate
by
difference
the
net
heat
produced
by
the
muscle.
In
Table
6
we
give
for
six
different
average
ratios
P/P0
the
sum
of
eight
individual
data
corresponding
approximately
to
that
load.
Admittedly
Fales'
experiments
were
not
designed
for
that
type
of
analysis
(three
missing
data
have
been
196

HEAT
OF
SHORTENING
IN
MUSCLE
interpolated),
but
fortunately
each
experiment
started
and
ended
with
an
isometric
series,
so
that
the
effect
of
load
and
shortening
on
the
heat
produced
can
be
fairly
estimated.
The
last line
of
Table
6
gives
the
difference
between
the
net
heat
produced
under
isotonic
conditions
and
the
average
isometric
heat.
Except
at
the
lightest
load,
more
heat
was
produced
in
isotonic
than
in
isometric
contractions.
As
information
con-
cerning
the
extent
of
shortening
is
lacking,
it
is
impossible
to
quantify
further,
but
the
main
effect
is
obvious:
after
a
series
of
five
cycles
of
con-
traction
and
relaxation,
when
the
load
moved
is
not
too
small,
a
true
calorimeter
records
a
net
thermal
effect
of
the
shortening.
Fales'
results
as
well
as
ours
concern
heat
production;
but
they
appear
to
have
a
chemical
counterpart
in
the
experiments
of
Jobsis
&
Duffield
(1967)
who
measured
by
fluorimetry
the
total
amount
of
disturbance
of
reduced
nicotinamide-adenine
dinucleotide,
during
the
period
of
aerobic
recovery
following
isotonic
twitches.
According
to
these
authors,
the
energy
utilization
is
determined
not
only
by
the
time
integral
of
the
force
development
and
by
the
mechanical
work,
but
also
by
the
time
integral
of
the
shortening
process.
Even
if
this
result
does
not
fit
exactly
with
the
original
concept
of
Hill
(1938),
it
points
in
the
same
direction.
The
negative
results
reported
by
some
authors
must
now
be
faced.
Carlson
et
al.
(1963)
did
not
find
any
evidence
of
a
net
thermal
effect
of
the
shortening
in
the
case
of
a
series
of
twitches;
but
as
already
suggested
by
Hill
(1964b),
the
fall
of
tension
associated
with
the
shortening
might
affect
the
duration
of
the
isotonic
twitches
and
so
alter
the
total
energy
output.
Recent
experiments
of
P.
Edman
(personal
communication)
showing
a
marked
decrease
of
the
active
state
by
an
initial
release
without
load
bring
direct
support
to
that
interpretation.
As
for
the
failure
to
detect
a
chemical
counterpart
of
the
heat
of
shorten-
ing,
different
explanations
are
possible.
First
of
all,
as
already
emphasized
by
Hill
(1966),
the
effect
looked
for
is
very
small
and
near
the
limits
of
chemical
detection.
In
our
experimental
conditions,
for
instance,
the
isometric
'short'
tetanus
gives
out
259
dimensionless
units
of
energy
(see
Table
2),
which
would
correspond
to
I
13
,umole/g
ATP
or
phosphoryl-
creatine
hydrolysed
(assuming
a
AH
of
10
kcal/mole);
to
this
basic
meta-
bolism,
shortening
would
add
up
to
0
13
gmole/g
for
the
extra
heat
and
about
the
same
amount
for
the
mechanical
work
performed
(see
Section
3
of
Results).
Different
authors
have
attempted
to
increase
the
magnitude
of
the
effect
either
by
working
on
series
of
twitches,
or
by
following
the
scheme
proposed
by
Hill
(1966).
We
have
already
said
why
the
use
of
twitches
is
objectionable.
As
for
the
design
originally
proposed
by
Hill
and
used
by
Kushmerick
et
al.
(1969),
it
is
open
to
the
following
criticism:
the
isometric
control
of
the
pair
of
muscles
produces
not
only
the
heat
taken
as
reference,
but
also
some
work
during
the
stretching
of
the
series
elastic
197

1X.
AUBERT
AND
J.
LEBACQ
elements.
This
work
has
no
equivalent
in
the
unloaded
isotonic
of
the
pair;
it
is
by
no
means
negligible
and
must
entail
a
supplement
of
chemical
expenditure.
As
shown
in
Table
6,
it is
precisely
in
the
case
of
unloaded
contraction
that
Fales'
experiments
do
not
show
any
thermal
effect
of
shortening.
2.
Why
is
the
thermal
effect
of
shortening
reduced
during
relaxation?
In
most
experiments
reported
here
the
supplement
of
heat
appearing
during
the
shortening
of
the
contractile
elements
is
partially
compensated
by
a
small
decrease
of
the
heat
produced
during
relaxation.
This
entails
a
reduction
of
15-25
%
of
the
net
effect
of
the
shortening.
The
reduction
is
most
conspicuous
in
the
pelvic
part
of
the
muscle,
where
it
reaches
an
average
of
37
%
of
the
effect
estimated
during
the
contraction
proper
(Fig.
3
and
Table
5).
The
difference
of
heat
production
in
the
two
parts
of
the
muscle
is
especially
large
during
relaxation.
It
is
probably
related
to
the
temporary
dissociation
of
length
and
tension
near
one
extremity
of
the
muscle
described
recently,
with
different
tech-
niques,
by
Cleworth
&
Edman
(1969)
and
by
Huxley
&
Simmons
(1970).
Whatever
its
cause,
it
limits
the
use
of
the
conventional
thermopile
for
the
estimation
of
an
accurate
balance
sheet
of
energy.
For
that
purpose,
the
integrating
thermopile
of
Wilkie
(1968)
or
the
gradient
layer
calorimeter
of
Fales
(1969)
are
more
appropriate.
But
these
instruments
are
too
slow
for
the
analysis
of
the
time
course
of
the
heat
production.
The
heterogeneity
of
response
of
the
muscle
along
its
length
complicates
the
evaluation
of
the
apparent
partial
reversal
of
the
heat
of
shortening;
it
does
not
explain
it.
On
the
other
hand
the
result
illustrated
in
Fig.
4
suggests
that
this
reduction
can
be
entirely
accounted
for
by
the
deficit
of
tension
following
an
active
shortening
(Table
1
and
Fig.
1).
As
relaxa-
tion
heat
appears
to
be
uniquely
determined
by
the
tension
at
the
end
of
stimulation,
any
factor
affecting
the
tension
should
modify
the
heat
of
relaxation
accordingly.
The
real
problem
is
thus
why
an
active
shortening
should
decrease
the
tension
developed
at
a
given
length.
No
explanation
is
presently
available.
In
conclusion,
when
a
muscle
shortens
actively
at
constant
velocity
during
contraction,
it
performs
work,
it
liberates
a
supplement
of
heat
and
the
tension
it
can
bear
at
the
shorter
length
is
reduced.
As
a
result
of
this
decrease
of
tension,
relaxation
heat
is
less
than
in
a
truly
isometric
con-
traction
at
the
same
final
length,
the
deficit
being
of
the
order
of
15-25
%
of
the
heat
of
shortening
measured
during
contraction.
The
latter
is
thus
not
part
of
a
cyclic
process,
which
would
be
reversed
during
relaxation;
it
must
involve
the
expenditure
of
free
energy.
If
no
equivalent
of
chemical
energy
is
found
under
the
form
of
ATP
or
phosphorylcreatine
hydrolysis,
other
types
of
chemical
reactions
must
be
looked
for.
198

HEAT
OF
SHORTENING
IN
MUSCLE
REFERENCES
ABBOTT,
B.
C.
&
Au-BERT,
X.
M.
(1952).
The
force
exerted
by
active
striated
muscle
during
and
after
change
of
length.
J.
Physiol.
117,
77-86.
ABBOTT,
B.
C.,
AUBERT,
X.
M.
&
HILL,
A.
V.
(1951).
The
absorption
of
work
by
a
muscle
stretched
during
a
twitch
or
a
short
tetanus.
Proc.
R.
Soc.
B
139,
86-104.
AUBERT,
X.
(1956).
In
Le
couplage
6nergetique
de
la
contraction
mu8culaire,
pp.
1-315.
Brussels:
Arscia.
CAIN,
D.
F.,
INFANTE,
A.
A.
&
DAvIEs,
R.
E.
(1962).
Adenosine
triphosphate
and
phosphorylcreatine
as
energy
supplies
for
single
contractions
of
working
muscles.
Nature,
Lond.
196,
214-217.
CARLSON,
F.
D.,
HARDY,
D.
J.
&
WILKIE,
D.
R.
(1963).
Total
energy
production
and
phosphocreatine
hydrolysis
in
the
isotonic
twitch.
J.
gen.
Phyaiol.
46,
851-882.
CLEWORTE,
D.
&
EDMAN,
K.
A.
P.
(1969).
Laser
diffraction
studies
on
single
skeletal
fibers.
Science,
N.Y.
263,
296-298.
DAvIEs,
R.
E.,
KusHMiRICK,
M.
J.
&
LARSON,
R.
E.
(1967).
ATP,
activation
and
the
heat
of
shortening
of
muscle.
Nature,
Lond.
214,
148-151.
FALES,
J.
T.
(1969).
Muscle
heat
production
and
work:
effect
of
varying
isotonic
load.
Am.
J.
Phy8iol.
216,
1184-1187.
FE:NN,
W.
0.
(1923).
A
quantitative
comparison
between
the
energy
liberated
and
the
work
performed
by
the
isolated
sartorius
muscle
of
the
frog.
J.
Phy8iol.
58,
175-203.
HILL,
A. V.
(1938).
The
heat
of
shortening
and
the
dynamic
constants of
muscle.
Proc.
R.
Soc.
B
126,
136-195.
HILL,
A.
V.
(1949).
The
heat
of
activation
and
the
heat
of
shortening
in
a
muscle
twitch.
Proc.
R.
Soc.
B
136,
195-211.
HILL,
A. V.
(1961).
The
heat
produced
after
the
last
shock
of
a
tetanus.
J.
Phyaiol.
159,
518-545.
HILL,
A.
V.
(1964a).
The
effect
of
load
on
the
heat
of
shortening
of
muscle.
Proc.
R.
Soc.
B
159,
297-318.
HILL,
A.
V.
(1964b).
The
effect
of
tension
in
prolonging
the
active
state
in
a
twitch.
Proc.
R.
Soc.
B
159,
589-595.
HILL,
A.
V.
(1964c).
The
variation
of
total
heat
production
in
a
twitch
with
velocity
of
shortening.
Proc.
R.
Soc.
B
159,
596-605.
HILL,
A.
V.
(1966).
A
further
challenge
to
biochemists.
Biochem.
Z.
345,
1-8.
HuxLEY,
A.
F.
&
SIMMONS,
R.
M.
(1970).
Rapid
'give'
and
the
tension
'shoulder
'
in
the
relaxation
of
frog
muscle
fibres.
J.
Physiol.
210,
32-33
P.
JOBSIS,
F.
F.
&
DUFFIELD,
J.
C.
(1967).
Force,
shortening
and
work
in
muscular
contraction:
relative
contribution
to
overall
energy
utilization.
Science,
N.
Y.
156,
1388-1392.
KusHMERICK,
M.
J.,
LARSON,
R.
E.
&
DAvIEs,
R.
E.
(1969).
The
chemical
energetics
of
muscle
contraction.
I.
Activation
heat,
heat
of
shortening
and
ATP
utilization
for
activation-relaxation
processes.
Proc.
R.
Soc.
B
174,
293-313.
LEBACQ,
J.
&
AUBERT,
X.
(1970).
Effet
du
raccourcissement
sur
la
production
de
chaleur
initiate
du
muscle
sartorius
de
'Rana
temporaria'.
Archs
int.
Physiol.
78,
561-563.
MAR1ACHAL,
G.
(1964).
In
Le
mgtabolieme
de
la
pho8phorylcreatine
et
de
l'adenosine
triphosphate
durant
la
contraction
musculaire,
pp.
1-184.
Brussels:
Arscia.
MOMMAERTS,
W.
F.
H.
M.,
SERAYDARIAN,
K.
&
MARACHAL,
G.
(1962).
Work
and
chemical
change
in
isotonic
muscular
contractions.
Biochim.
biophy8.
Acta
57,
1-12.
199

200
X.
AUBERT
AND
J.
LEBACQ
MOMMAERTS,
W.
F.
H.
M.
(1970).
What
is
the
Fenn-effect?
Naturwi88en8chaften
57,
326-330.
PRINGLE
J.
W.
S.
(1960).
Models
of
muscle.
Symp.
Soc.
exp.
Biol.
15,
41-68.
WILKIE,
D.
R.
(1968).
Heat,
work
and
phosphorylereatine
break-down
in
muscle.
J.
Physiol.
195,
157-183.
WOLEDGE,
R.
C.
(1961).
The
thermoelastic
effect
of
change
of
tension
in
active
muscle.
J.
Phy8iol.
155,
187-208.